Observational Geodynamics
Convection in the mantle is a complex process. Various physical parameters exist that have a critical impact on it. To understand which parameters are more important than others and to determine which values they assume, mantle convection models must be tested. Fortunately, there is a growing number of geodynamically relevant observations for the solid Earth. They are pursued vigorously in our group and include:
Seismic wave propagation in geodynamic Earth models
The seismic signature of our planet is an essential reference for geodynamic models. Over the past few decades, seismological studies have revealed distinct physical structures in Earth’s mantle that entail a plethora of geophysical implications. While seismology provides data and models of the present-day state of Earth, the exact nature and evolution of the mantle can only be investigated in a dynamic flow model. Understanding the link between seismology and geodynamics in a quantitative manner thus is one of our main areas of research. To bridge the gap between geophysical hypotheses and seismic observations, we follow a multidisciplinary approach:
- Mantle circulation is simulated at high resolution to predict the present-day mantle temperature field.
- Thermodynamic models of mantle mineralogy are used to convert temperatures to seismic velocities or vice versa. This ensures a physically consistent seismic representation of the geodynamic model, which is a prerequisite to
- Predict synthetic seismic data on a global scale, foremost full-waveform seismograms from numerical 3-D wave propagation simulations.
- Synthetic data can be compared to seismic observations, which allows for an explicit testing of the geodynamic mantle circulation model. Alternatively, it is possible to compare geodynamic models directly to 3D-images of the mantle obtained by seismic tomography. Such comparisons are only meaningful if the limited resolution of seismic tomography and the associated uncertainties are accounted for. In our studies, this is done by so-called "tomographic filtering" of the geodynamic mantle structures. This way, we can look at our models with the same “lense” that seismic tomography uses to probe the real Earth.
In the context of our seismic forward modelling, our further interests in the research area between seismology and geodynamics include the role of phase transitions for global mantle flow and their effect on seismic data, normal mode constraints on mantle properties, as well as the prediction of seismic body-wave traveltime residuals in our geodynamic models with ray-theory and finite-frequency sensitivity kernels.
Current and past plate motions
Many surface processes, including motions of the Earth’s tectonic plates, can be related to underlying mantle circulation. Geological manifestations that are evidence of this, can be found across the Earth’s surface, such as the Andean mountain range in South America and the elevated topography of the African continent. In turn, these surface features have an impact on mantle convection, modulating and organizing its convective planform.
It is possible to reconstruct plate motions based on observations of the ocean floor’s age, its magnetization and the occurrence of fracture zones. An accurate representation of these observations is essential to study plate tectonics and mantle dynamics. An outstanding characteristic of past plate motions is the record of rapid (on the order of a few million years) temporal plate motion variations. These changes occur on short time scales relative to the time it takes for the large-scale structure associated with mantle buoyancy to evolve. Past and present-day plate motions are increasingly well mapped. They allow geodynamicists to connect structures in the mantle to the history of subduction and to link plate tectonics to mass motion driven by mantle convection. A difficulty arises from the superposition of sublithospheric and shallow plate-boundary forces. This hinders our interpretation of plate motions solely in terms of forces related to mantle convection or lithosphere dynamics. In particular, our efforts in this context focus on (i) simulating the impact of the mantle time-evolving buoyancy field on plate motions; and (ii) exploring the dynamics of mantle and lithosphere interactions by using the record of plate motions as a reference to validate parameter choices in numerical simulations.
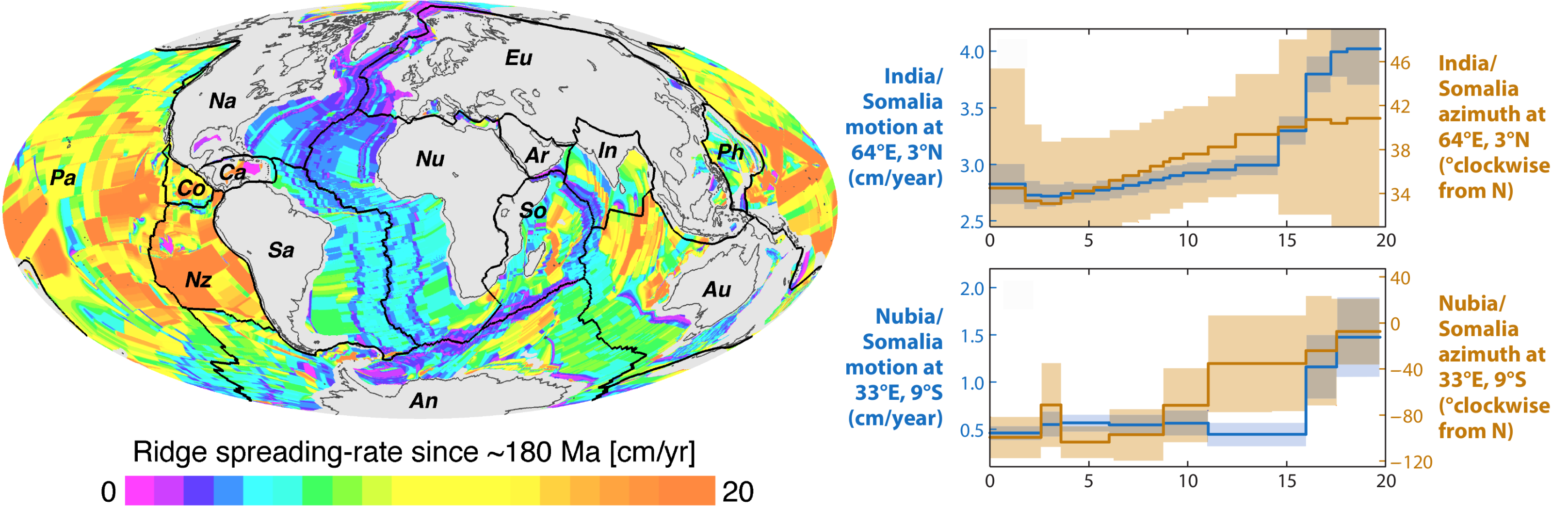
Mantle-flow-induced dynamic topography
It is well known that plate tectonics is intrinsically linked with large-scale convective forces in the mantle. However, geologic observations also document the occurrence of significant vertical surface motions that cannot be explained by eustatic sea level changes or isostatic considerations alone. Flow in the underlying mantle is the likely cause that governs these surface deflections. This is known as "dynamic topography".
Important observations that allow us to understand mantle-flow-induced dynamic topography and its temporal evolution can be found in the geologic record, specifically the distribution of sediments. Continent scale sediment distributions are characterized by unconformities (absence of a stratigraphic unit) owing to non-deposition or erosion of sedimentary layers. These surfaces, also known as hiatal surfaces, can be mapped through geological times for geodynamic purposes. This allows us to get proxies for paleotopography. The latter relate to changes in the vertical motion of the lithosphere at interregional scales. Other techniques that yield valuable constraints on various timescales are, for example, GPS measurements, drainage analysis, mapping of paleosurfaces, thermochronology and more (for a comprehensive overview, see Hoggard et al. 2020, Observational estimates of dynamic topography through space and time).
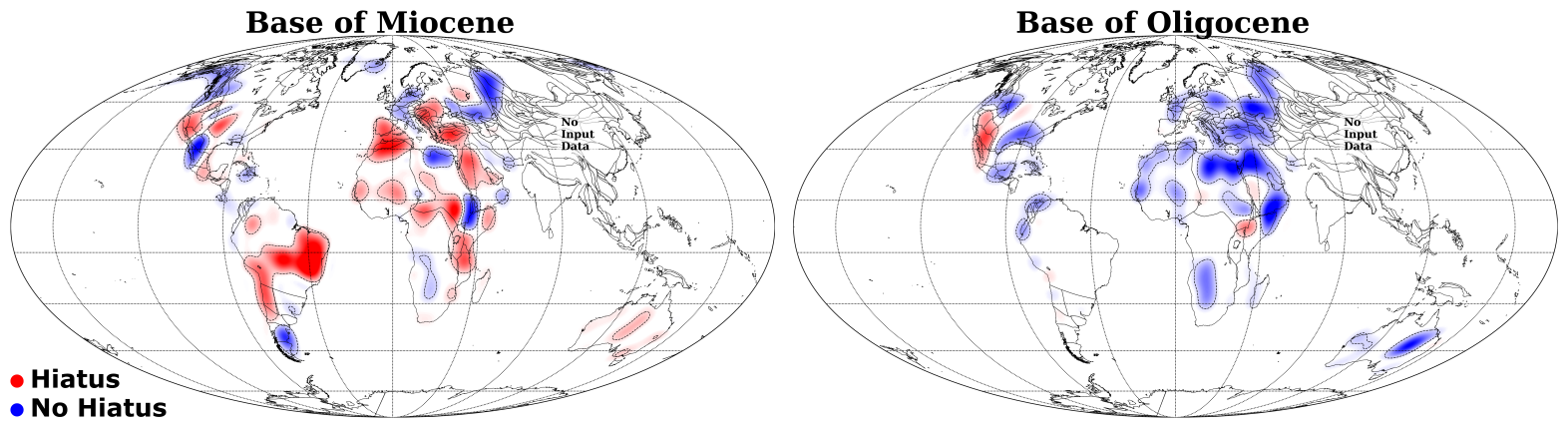
Estimates of time-dependent dynamic topography provide a new and powerful class of geodynamically relevant observations, which we compile for comparison with next-generation Earth models.
Geodetic constraints
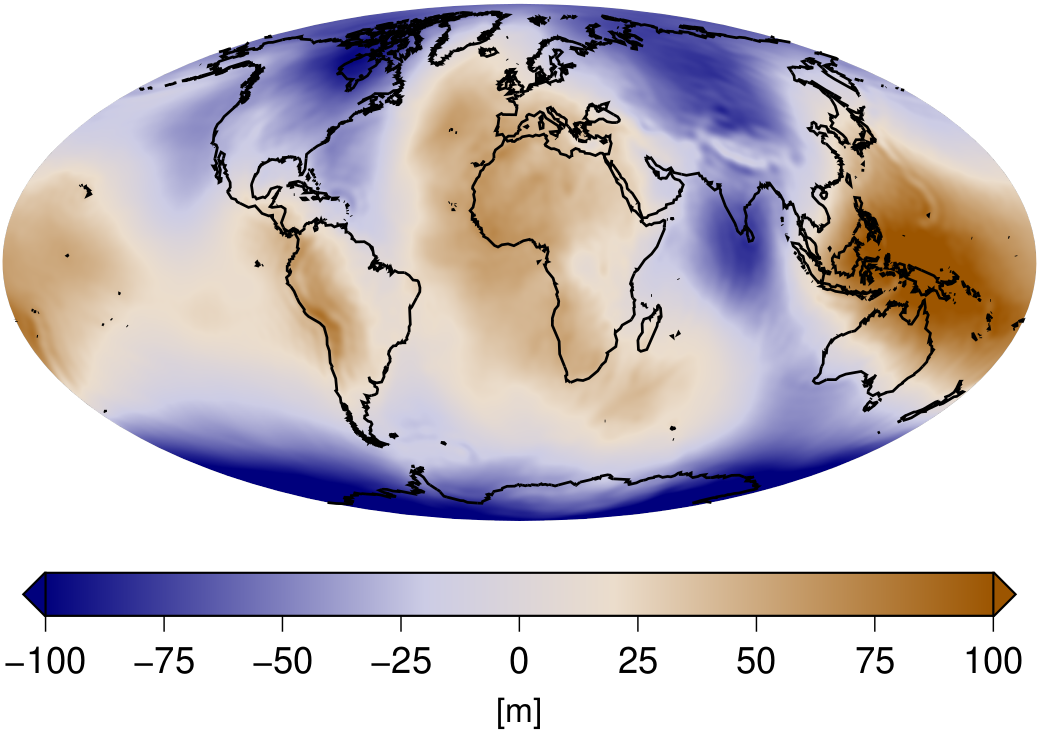
By definition, mantle circulation leads to a redistribution of masses. It thus has a dominant influence on the internal density structure of the Earth. The associated gravity field provides a valuable data set to constrain properties of the Earth’s interior. Moreover, modern satellite missions, like e.g. GRACE, GOCE and GRACE-FO, are capable of providing global data coverage and highly accurate measurements of the Earth’s present-day gravitational structure.
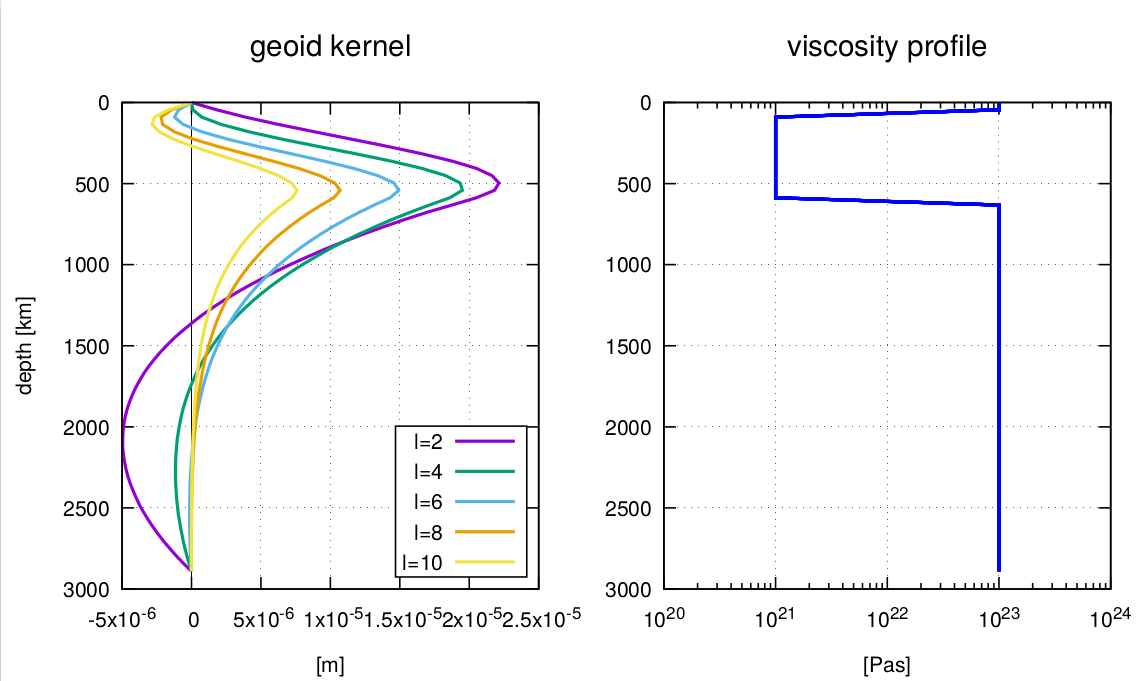
Unfortunately, a direct determination of the Earth’s interior density structure, relying solely on gravity data, is impossible. Nevertheless, in combination with seismic tomography, gravity signals provide a powerful tool to constrain the radial viscosity structure of the mantle. To this end, so-called "geoid kernels" are well known. They represent the gravitational response of a unit density anomaly at a certain depth level, including effects from flow-induced dynamic topography. The kernels are highly sensitive to the viscosity stratification inside the Earth’s mantle. Exploiting this sensitivity, one can show that the observed gravity field can only be explained with a viscosity profile that includes a low-viscosity channel in the upper mantle, the so-called "asthenosphere".
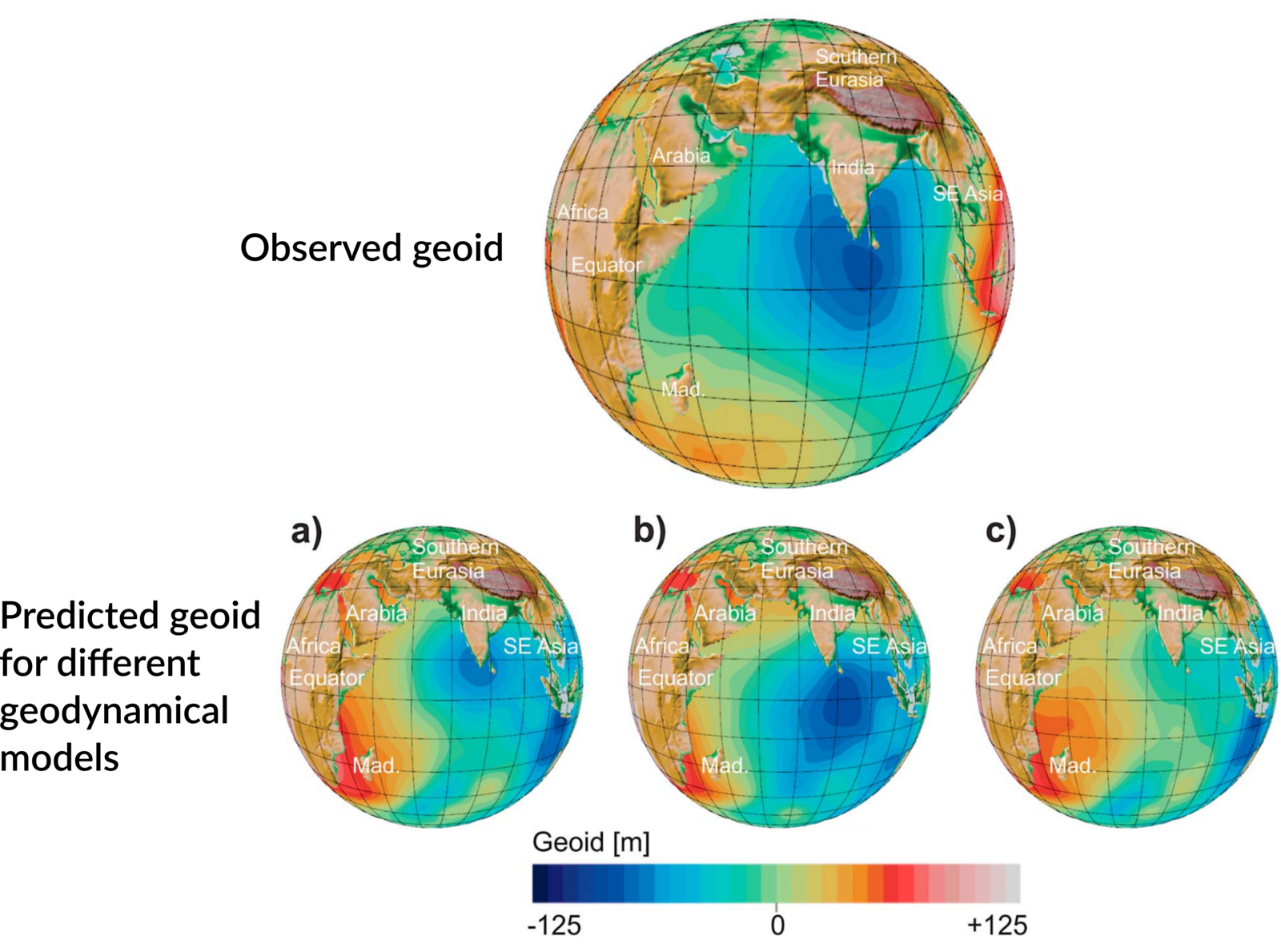
This method has one main drawback: The viscosity profile cannot be determined uniquely. To overcome this ambiguity, one has to turn to time-dependent Earth models. This naturally motivates the development of mantle circulation models.